Forró Group
Scope
The Forró research group is seeking to build bridges between the physical, chemical, and biological sciences, the hard and soft matter themes. For example, a strong program in crystal and film growth and characterization is crucial for our studies of correlated electron materials; serious research on nanostructures gives us tools to address important issues on biomolecules; similarly, all improvements in spectromicroscopy instrumentation enhance our research on biological samples. Ultimately, biomedical imaging and cell-research benefit from the progress in many sub-areas of condensed matter physics.
Novel Quantum Materials
It is generally believed that the simple charge modulation employed in MOSFET electronics will not be sufficient for future electronic devices. Controlling and manipulating new degrees of freedom, like spin and orbits will play major role in the new generation of electronic materials like organic conductors, manganites, vanadates and cuprates. Therefore the understanding of the basic properties of such systems is primordial. Their engineering could have a significant impact on the electronics of the twenty first century and some of them are already present in new technologies.
It turns out that in most of these materials the electronic mean free path is very short, often even shorter than the lattice spacing. In other words, despite the metallic-like temperature dependence of the resistivity, strictly speaking they do not qualify for metals and they are very often called bad metals. Our goal is to investigate the bad metallicity by transport and magnetotransport studies in wide pressure (up to 20 GPa) and temperature (50 mK - 1000 K) ranges. The lattice compression can vary the electron-phonon coupling, the screening of the electron-electron interactions, alter the exchange interaction, suppress low-dimensional fluctuations, etc. We are confident that in some cases high pressures can tune the transition from a bad metal to a good metal (or from non-Fermi liquid to a Fermi liquid), thus contributing towards the understanding of this peculiar state. Our research in novel electronic materials is described in this general context.
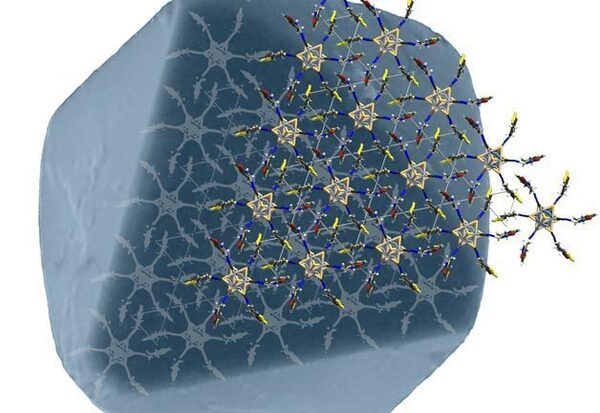
Graphene and its derivatives
Graphene is interesting for basic science, because it has a highly atypical band structure. It has raised notions like massless Dirac fermions, pseudospin, Berry phase, Klein paradox, Zitterbewegung and the spectacular manifestation of the integer and fractional quantum Hall effects.
But beyond the beauty for the basic research, graphene is even more interesting for applications. It is considered as a promising building block for prospective electronic technologies among which is spintronics. This is partially based on the high mobility of charge carriers and on a low spin-orbit coupling which is synonymous with a long spin lifetime. The goal of our research is to study the magnetic properties of graphene, and to extract the spin relaxation time by Electron Spin Resonance (ESR) measurements. It turns out that it is not so easy to pin down this quantity, because it depends on the environment of graphene, on the substrate by which it is supported, and last but not least, on the production method of it. One of the non-negligible goals of our work is to identify which production route of graphene gives the appropriate quality for spintronic applications and to find the intrinsic spin life-time of a real material.
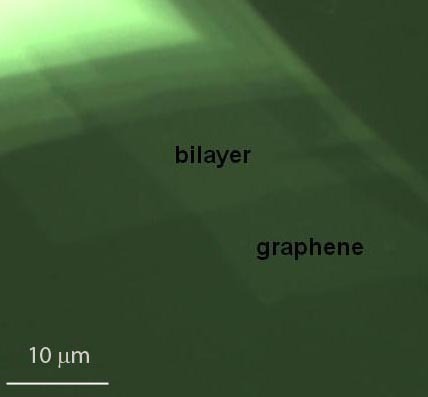
Titanium dioxide
Titanium dioxides is an attractive material due to its potential applications in optoelectronic devices (such as flat panel displays, organic light-emitting diodes), and especially in dye sensitized solar cells. Although numerous new phenomena have been observed in this system, its electronic properties still represent a few puzzles. Comprehending and engineering the conductivity of this material would be an important step towards a broader range of applications.
On the fundamental side, there is continuous interest in the charge propagation in polar materials like TiO2 where mobile charge carriers are introduced by doping and show a polaronic character. At one end, the strong interaction between conduction electrons and phonons leads to the formation of small polarons and carrier localization through self-trapping. For weaker coupling, charge carriers remain mobile but have an enhanced effective mass meff, as they travel through the crystal as large polarons. These quasiparticles are particularly attractive, because they can be studied by conventional transport measurements which have relevance in electronic applications.
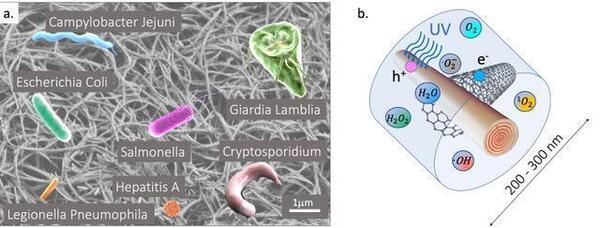
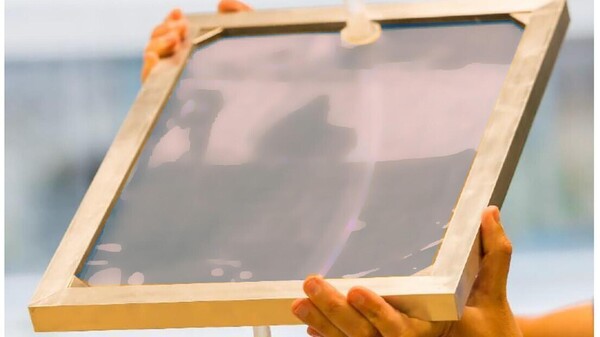